With their target completion date just a year and a half away, scientists and technicians at the National Ignition Facility (NIF) are quickening their pace to install and test the rest of NIF’s 192 lasers and prepare for a new round of preliminary experiments in 2008.This is a report from Lawrence Livermore National Laboratory (LLNL) official web site:
Ninety-six NIF beamlines have been fired together for the first time, with “excellent” control system and laser stability, according to NIF & Photon Science Principal Associate Director Ed Moses. Last month the facility’s injection laser systems, which initiate the laser pulses, were fired for 144 beamlines.
“A total infrared energy of more than 2.5 megajoules has now been fired,” Moses said. “This is more than 40 times what the Nova laser (NIF’s predecessor) typically operated at the time it was the world's largest laser.”
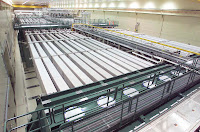
The first of the facility’s two 96-beam laser bays was commissioned at the end of July. Each of the 96 beams fired an infrared output energy of about 22,000 joules, more than enough to meet NIF’s operational and performance requirements. Since then six more eight-beam “bundles” are being commissioned in the second laser bay, and three of these bundles have been operationally qualified.
Overall commissioning of the NIF beamlines is scheduled for 2009.
The laser shots last about 25 billionths of a second, a tiny fraction of the time it takes to blink an eye. Firing the beams requires operation of 2,300 high-quality optics and instrumentation modules and nearly 400 computers running a million lines of control system code.
The tests measure the quality of each beam’s spatial profile and temporal pulse shape. Even though each shot is exceedingly short in time, its energy output and frequency is designed to vary significantly throughout its duration depending on the type of experiments being conducted.
Meanwhile, data gathered from experiments conducted at NIF in 2003-2004 have enabled sophisticated computer simulations that confirm NIF’s ability to reach the energy levels and beam quality required to produce the world’s first demonstration of inertial confinement fusion.
The “NIF Early Light” experiments included four shots using four laser beams at high energy on a full-scale target for the first time. Simulations of the experiments on LLNL’s world-class supercomputers matched the actual experimental data to an unprecedented degree. The experiments and simulations indicate that NIF’s laser beams will propagate effectively in plasma-filled targets designed to achieve fusion ignition and thermonuclear burn.
NIF experiments next year will focus 96 beams on a gold hohlraum (the eraser-sized capsule containing the fusion target) filled with a light gas mixture. Dubbed “Eos” for the Greek goddess of dawn, the experiments will use the first set of beams from the completed laser bay, traveling to the center of the ten-meter diameter target chamber. They are designed to help validate key aspects of the full-scale ignition campaign that begins in 2010.