An international team that includes University of British Columbia physicists has used ultra-fast laser pulses to identify the microscopic interactions that drive high-temperature superconductivity.
In the experiment, to be outlined this Friday in the journal Science, electrons in a prototypical copper-oxide superconductor were excited by extremely short 100-femtosecond laser pulses.
As the material's electrons relax back to an equilibrium state, they release their excess energy via deformation of the superconductor's atomic lattice (phonons) or perturbation of its magnetic correlations (spin fluctuations).
The researchers were able to capture very fine grained data on the speed of the relaxation process and its influence on the properties of the superconducting system, showing that the high-critical temperature of these compounds can be accounted for by purely electronic (magnetic) processes.
"This new technique offers us our best window yet on the interactions that govern the formation of these elusive superconducting properties--both across time and across a wide range of characteristic energies," says UBC Associate Professor Andrea Damascelli, Canada Research Chair in Electronic Structure of Solids with the Department of Physics and Astronomy and the UBC Quantum Matter Institute.
"We're now able to begin to disentangle the different interactions that contribute to this fascinating behavior."
Superconductivity--the phenomenon of conducting electricity with no resistance--occurs in some materials at very low temperatures. High-temperature cuprate superconductors are capable of conducting electricity without resistance at temperatures as high as -140 degrees Celsius.
The key mechanism that allows the carriers to flow without resistance in superconductors stems from an effective pairing between electrons. In conventional metallic superconductors, this pairing mechanism is well understood as phonon-mediated. In copper-oxides, the nature of the low-resistance interaction between the electrons has remained a mystery.
"This breakthrough in the understanding of the puzzling properties of copper-oxides paves the way to finally solving the mystery of high-temperature superconductivity and revealing the key knobs for engineering new superconducting materials with even higher transition temperatures," says the paper's lead author Claudio Giannetti, a researcher with Italy's Università Cattolica del Sacro Cuore and visiting professor at UBC's Quantum Matter Institute.
The international collaboration also involved contributions from Japanese, Swiss and American researchers.
More information: "Disentangling the Electronic and Phononic Glue in a High-Tc Superconductor," by S. Dal Conte, et al., Science.
Thursday, March 29, 2012
Thursday, March 22, 2012
NIF facility fires record laser shot into target chamber
(PhysOrg.com) -- The National Ignition Facility (NIF) at Lawrence Livermore National Laboratory in California has set a new record for a laser shot. This past week, its combined 192 lasers fired a single 1.875-megajoule shot into an empty test chamber. After passing through the last of its focusing lens, the shot reached 2.03 megajoules, making it the first 2 megajoule ultraviolet laser.
Prior to this achievement, the most the facility had managed to coax out of the laser, the world’s largest, was 1.6 megajoules. Also, the new record shows that the NIF laser is capable of producing more than it was designed for, which was 1.8 megajoules. It also proved that it was capable of doing so without damaging its parts, allowing for another shot a day and a half later, which is important, because one of the goals for the laser is to get it to fire off shots at 15 per second eventually. That’s what researchers think will be needed to produce power economically from the laser system.
The ultimate goal of the NIF is to figure out a way to use a laser to produce nuclear fusion in a way that gets more energy out than is put in. Currently, that goal is still a ways off. Thus far, engineers at the project haven't even reached the break-even or ignition point, though they expect that to occur sometime this year. Tweaking the laser to produce more than it was designed for is a step in that direction. The NIF facility was designed to produce a fusion reaction by imploding hydrogen isotope pellets using the huge laser. To that end, the team has made steady progress. When the project first began eighteen months ago, it had just one percent of conditions in place that are believed necessary to achieve the ignition point. They have improved that mark to ten percent and it’s because the pace has picked up dramatically in recent months that they believe they will achieve the ignition point sometime over the next six months, which is when the original ignition campaign was slated to end.
Because the facility is funded by the US nuclear weapons complex, there has been debate about whether it would ever be used to prove or disprove the idea that lasers could be used to create nuclear fusion to produce electric power. Having the laser break records doesn’t really resolve that argument in the short term, but it might in the long run if it does eventually show that electricity could be created economically using such a process.
More information: The National Ignition Facility (NIF): https://lasers.llnl.gov/
Prior to this achievement, the most the facility had managed to coax out of the laser, the world’s largest, was 1.6 megajoules. Also, the new record shows that the NIF laser is capable of producing more than it was designed for, which was 1.8 megajoules. It also proved that it was capable of doing so without damaging its parts, allowing for another shot a day and a half later, which is important, because one of the goals for the laser is to get it to fire off shots at 15 per second eventually. That’s what researchers think will be needed to produce power economically from the laser system.
The ultimate goal of the NIF is to figure out a way to use a laser to produce nuclear fusion in a way that gets more energy out than is put in. Currently, that goal is still a ways off. Thus far, engineers at the project haven't even reached the break-even or ignition point, though they expect that to occur sometime this year. Tweaking the laser to produce more than it was designed for is a step in that direction. The NIF facility was designed to produce a fusion reaction by imploding hydrogen isotope pellets using the huge laser. To that end, the team has made steady progress. When the project first began eighteen months ago, it had just one percent of conditions in place that are believed necessary to achieve the ignition point. They have improved that mark to ten percent and it’s because the pace has picked up dramatically in recent months that they believe they will achieve the ignition point sometime over the next six months, which is when the original ignition campaign was slated to end.
Because the facility is funded by the US nuclear weapons complex, there has been debate about whether it would ever be used to prove or disprove the idea that lasers could be used to create nuclear fusion to produce electric power. Having the laser break records doesn’t really resolve that argument in the short term, but it might in the long run if it does eventually show that electricity could be created economically using such a process.
More information: The National Ignition Facility (NIF): https://lasers.llnl.gov/
Thursday, March 15, 2012
Scientists reveal inner workings of magnets, a finding that could lead to faster computers
Using a light source that creates X-ray pulses only one quadrillionth of a second in duration, the Boulder team was able to observe how magnetism in nickel and iron atoms works, and they found that each metal behaves differently. One quadrillionth of a second is a million times faster than one billionth of a second.
The results of the study were published online this week by the Proceedings of the National Academy of Sciences. Six of the study’s 19 co-authors are located at CU-Boulder.
Many technology experts believe that next-generation computer disk drives will use optically-assisted magnetic recording to achieve much higher drive capacities, according to NIST scientist Tom Silva, who worked with CU-Boulder physics professors Margaret Murnane and Henry Kapteyn on the research. However, many questions remain about how the delivery of optical energy to the magnetic system can be optimized for maximum drive performance. And this finding could help researchers answer some of their questions.
“The discovery that iron and nickel are fundamentally different in their interaction with light at ultrafast time scales suggests that the magnetic alloys in hard drives could be engineered to enhance the delivery of the optical energy to the spin system,” Silva said.
Magnetism exists because all of the “spins” in a magnet -- each of which is like a very small bar magnet with a north and south pole -- are lined up to point in the same direction, much like members of a marching band who are moving in unison, explained Murnane, who also is a fellow of JILA, a joint institute of CU-Boulder and NIST.
“The powerful laser pulse scrambles the magnetic spins in the metal, as if the members of the marching band started moving in different directions across the football field, causing the magnetization to rapidly disappear within a mere fifty quadrillionths of a second, a process known as ultrafast demagnetization,” Murnane said.
While ultrafast demagnetization has been a well-known phenomenon since its discovery in 1996, the CU and NIST researchers saw for the first time that different kinds of spins in metal scramble on different time scales. Until now, it was assumed that all the spins in a metal alloy behaved in the same way due to a powerful quantum mechanical effect known as the exchange interaction, which lines up all the individual spins in the same direction.
“What we have seen for the first time is that the iron spins and the nickel spins react to light in different ways, with the iron spins being mixed up by light much more readily than the nickel spins,” said Silva. “In the end, the exchange interaction still pulls the two spin systems back into synchronization after a few quadrillionths of a second. Seeing such a difference was only possible by taking advantage of the extremely fast X-ray technology developed at the University of Colorado and elsewhere.”
The laser technology used in the experiment, known as “high harmonic generation,” can generate laser-like beams of X-rays that span a wide portion of the electromagnetic spectrum, including the spectral region where nickel and iron interact very strongly with X-rays.
The results of the study were published online this week by the Proceedings of the National Academy of Sciences. Six of the study’s 19 co-authors are located at CU-Boulder.
Many technology experts believe that next-generation computer disk drives will use optically-assisted magnetic recording to achieve much higher drive capacities, according to NIST scientist Tom Silva, who worked with CU-Boulder physics professors Margaret Murnane and Henry Kapteyn on the research. However, many questions remain about how the delivery of optical energy to the magnetic system can be optimized for maximum drive performance. And this finding could help researchers answer some of their questions.
“The discovery that iron and nickel are fundamentally different in their interaction with light at ultrafast time scales suggests that the magnetic alloys in hard drives could be engineered to enhance the delivery of the optical energy to the spin system,” Silva said.
Magnetism exists because all of the “spins” in a magnet -- each of which is like a very small bar magnet with a north and south pole -- are lined up to point in the same direction, much like members of a marching band who are moving in unison, explained Murnane, who also is a fellow of JILA, a joint institute of CU-Boulder and NIST.
“The powerful laser pulse scrambles the magnetic spins in the metal, as if the members of the marching band started moving in different directions across the football field, causing the magnetization to rapidly disappear within a mere fifty quadrillionths of a second, a process known as ultrafast demagnetization,” Murnane said.
While ultrafast demagnetization has been a well-known phenomenon since its discovery in 1996, the CU and NIST researchers saw for the first time that different kinds of spins in metal scramble on different time scales. Until now, it was assumed that all the spins in a metal alloy behaved in the same way due to a powerful quantum mechanical effect known as the exchange interaction, which lines up all the individual spins in the same direction.
“What we have seen for the first time is that the iron spins and the nickel spins react to light in different ways, with the iron spins being mixed up by light much more readily than the nickel spins,” said Silva. “In the end, the exchange interaction still pulls the two spin systems back into synchronization after a few quadrillionths of a second. Seeing such a difference was only possible by taking advantage of the extremely fast X-ray technology developed at the University of Colorado and elsewhere.”
The laser technology used in the experiment, known as “high harmonic generation,” can generate laser-like beams of X-rays that span a wide portion of the electromagnetic spectrum, including the spectral region where nickel and iron interact very strongly with X-rays.
Saturday, March 10, 2012
Making sharper X-rays
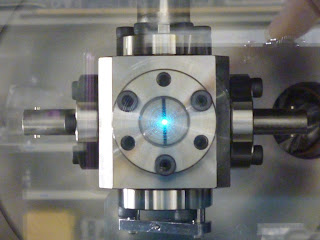
A variety of imaging technologies rely on light with short wavelengths because it allows very small structures to be resolved. However, light sources which produce short, extreme ultraviolet or x-ray wavelengths often have unstable emission wavelength and timing. Now, by illuminating a gas with a powerful laser, a research team in Japan has demonstrated a light source that may solve many of these problems. The research was published by Mitsuru Nagasono, from the RIKEN SPring-8 Center in Harima, and his colleagues from RIKEN and three other institutes.
More information: Nagasono, M., et al. Observation of free-electron-laser-induced collective spontaneous emission (superfluorescence). Physical Review Letters 107, 193603 (2011).
Friday, March 09, 2012
Femtolaser Pulse Creates 3-D Nanostructures
A new fabrication process using femtosecond lasers creates 3-D nanostructures in materials, an essential step toward creating invisibility cloaks and other advanced materials that bend light in unusual ways.
Researchers in Eric Mazur's laboratory at the Harvard School of Engineering and Science (SEAS) fired a femtosecond laser, which releases incredibly bright flashes of light that last 5 x 10^-14, s, at a glass slide coated with a mixture of silver nitrate, water and PVP, a water-soluble polymer. The laser blast changes the electrical, physical and optical properties of the slide, and photoreduces the silver ions on the slide into nanocrystals of silver metal suspended on the polymer.
Previous attempts to create a 3-D structure failed because the coating was not quite right. When the researchers used only the silver nitrate and water, there was no lattice support for the silver atoms, they said.
"Normally, when people use femtosecond lasers in fabrication, they’re creating a woodpile structure: something stacked on something else, being supported by something else. If you want to make an array of silver dots, however, they can’t float in space," Mazur said. Ethanol and the PVP polymer were added to the solution to provide support to the structure, but reactions were fast and uncontrollable. Removing the ethanol solved the problem entirely.
"What was most surprising about it was how simple it is. It was a matter of using less," Mazur said.
The new fabrication process advances nanoscale metal lithography into three dimensions, and does it at a resolution high enough to be practical for metamaterials.
"This work demonstrates that we can create silver dots that are disconnected in X, Y, and Z," said Kevin Vora, a graduate student working on the project. "There’s no other technique that feasibly allows you to do that."
The work, which was supported by the Air Force Office of Scientific Research, is described in Applied Physics Letters.
Researchers in Eric Mazur's laboratory at the Harvard School of Engineering and Science (SEAS) fired a femtosecond laser, which releases incredibly bright flashes of light that last 5 x 10^-14, s, at a glass slide coated with a mixture of silver nitrate, water and PVP, a water-soluble polymer. The laser blast changes the electrical, physical and optical properties of the slide, and photoreduces the silver ions on the slide into nanocrystals of silver metal suspended on the polymer.
Previous attempts to create a 3-D structure failed because the coating was not quite right. When the researchers used only the silver nitrate and water, there was no lattice support for the silver atoms, they said.
"Normally, when people use femtosecond lasers in fabrication, they’re creating a woodpile structure: something stacked on something else, being supported by something else. If you want to make an array of silver dots, however, they can’t float in space," Mazur said. Ethanol and the PVP polymer were added to the solution to provide support to the structure, but reactions were fast and uncontrollable. Removing the ethanol solved the problem entirely.
"What was most surprising about it was how simple it is. It was a matter of using less," Mazur said.
The new fabrication process advances nanoscale metal lithography into three dimensions, and does it at a resolution high enough to be practical for metamaterials.
"This work demonstrates that we can create silver dots that are disconnected in X, Y, and Z," said Kevin Vora, a graduate student working on the project. "There’s no other technique that feasibly allows you to do that."
The work, which was supported by the Air Force Office of Scientific Research, is described in Applied Physics Letters.
Thursday, March 08, 2012
First-ever images of atoms moving in a molecule captured
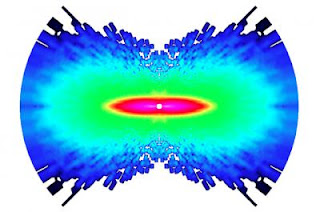
Key to the experiment, which appears in this week's issue of the journal Nature, is the researchers' use of the energy of a molecule's own electron as a kind of "flash bulb" to illuminate the molecular motion.
The team used ultrafast laser pulses to knock one electron out of its natural orbit in a molecule. The electron then fell back toward the molecule scattered off of it, analogous to the way a flash of light scatters around an object, or a water ripple scatters in a pond.
Principal investigator Louis DiMauro of Ohio State University said that the feat marks a first step toward not only observing chemical reactions, but also controlling them on an atomic scale.
"Through these experiments, we realized that we can control the quantum trajectory of the electron when it comes back to the molecule, by adjusting the laser that launches it," said DiMauro, who is a professor of physics at Ohio State. "The next step will be to see if we can steer the electron in just the right way to actually control a chemical reaction."
A standard technique for imaging a still object involves shooting the object with an electron beam – bombarding it with millions of electrons per second. The researchers' new single-electron quantum approach allowed them to image rapid molecular motion, based on theoretical developments by the paper's coauthors at Kansas State University.
A technique called laser induced electron diffraction (LIED) is commonly used in surface science to study solid materials. Here, the researchers used it to study the movement of atoms in a single molecule.
The molecules they chose to study were simple ones: nitrogen, or N2, and oxygen, or O2. N2 and O2 are common atmospheric gases, and scientists already know every detail of their structure, so these two very basic molecules made a good test case for the LIED method.
In each case, the researchers hit the molecule with laser light pulses of 50 femtoseconds, or quadrillionths of a second. They were able to knock a single electron out of the outer shell of the molecule and detect the scattered signal of the electron as it re-collided with the molecule.
DiMauro and Ohio State postdoctoral researcher Cosmin Blaga likened the scattered electron signal to the diffraction pattern that light forms when it passes through slits. Given only the diffraction pattern, scientists can reconstruct the size and shape of the slits. In this case, given the diffraction pattern of the electron, the physicists reconstructed the size and shape of the molecule – that is, the locations of the constituent atoms' nuclei.
The key, explained Blaga, is that during the brief span of time between when the electron is knocked out of the molecule and when it re-collides, the atoms in the molecules have moved. The LIED method can capture this movement, "similar to making a movie of the quantum world," he added.
Beyond its potential for controlling chemical reactions, the technique offers a new tool to study the structure and dynamics of matter, he said. "Ultimately, we want to really understand how chemical reactions take place. So, long-term, there would be applications in materials science and even chemical manufacturing."
"You could use this to study individual atoms," DiMauro added, "but the greater impact to science will come when we can study reactions between more complex molecules. Looking at two atoms – that's a long way from studying a more interesting molecule like a protein."
Subscribe to:
Posts (Atom)