Microchannels are critical to cost and efficiency because they are needed to interconnect a series of solar panels into an array capable of generating usable amounts of power, said Yung Shin, a professor of mechanical engineering and director of Purdue University's Center for Laser-Based Manufacturing. Conventional scribing methods, which create the channels mechanically with a stylus, are slow and expensive and produce imperfect channels, impeding solar cells' performance.
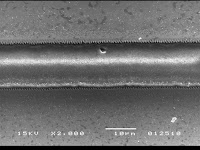
"Production costs of solar cells have been greatly reduced by making them out of thin films instead of wafers, but it is difficult to create high-quality microchannels in these thin films," Shin said. "The mechanical scribing methods in commercial use do not create high-quality, well-defined channels. Although laser scribing has been studied extensively, until now we haven't been able to precisely control lasers to accurately create the microchannels to the exacting specifications required."
"The efficiency of solar cells depends largely on how accurate your scribing of microchannels is," Shin said. "If they are made as accurately as possible, efficiency goes up."
The work, funded by a three-year, $425,000 grant from the National Science Foundation, is led by Shin and Gary Cheng, an associate professor of industrial engineering. A research paper demonstrating the feasibility of the technique was published in Proceedings of the 2011 NSF Engineering Research and Innovation Conference in January. The paper was written by Shin, Cheng and graduate students Wenqian Hu, Martin Yi Zhang and Seunghyun Lee.
Research results have shown that the fast-pulsing laser accurately formed microchannels with precise depths and sharp boundaries. The laser pulses last only a matter of picoseconds. Because the pulses are so fleeting, the laser does not cause heat damage to the thin film, instead removing material in precise patterns in a process called cold ablation.
"It creates very clean microchannels on the surface of each layer," Shin said. "You can do this at very high speed, meters per second, which is not possible with a mechanical scribe. This is very tricky because the laser must be precisely controlled so that it penetrates only one layer of the thin film at a time, and the layers are extremely thin. You can do that with this kind of laser because you have a very precise control of the depth, to about 10 to 20 nanometers."
Traditional solar cells are usually flat and rigid, but emerging thin-film solar cells are flexible, allowing them to be used as rooftop shingles and tiles or building facades, or as the glazing for skylights. Thin-film solar cells account for about 20 percent of the photovoltaic market globally in terms of watts generated and are expected to account for 31 percent by 2013.
The researchers plan to establish the scientific basis for the laser ablation technique by the end of the three-year period. The work is funded through NSF’s Civil Mechanical and Manufacturing Innovation division.
For more information, visit: www.purdue.edu