Wednesday, April 18, 2012
Looking through the glass transition on an ultrafast timescale
Glass is one of civilization's most valuable and versatile materials. To scientists, it's also one of the most intriguing, because it displays properties of both solids and liquids.
Glass is a non-crystalline solid that transforms into a liquid when it's heated to the so-called glass-transition temperature. When glass approaches this critical temperature—which is between 970 to 1,100 degrees Fahrenheit for the most common type of glass—it is simultaneously composed of fluid, flowing regions and solid-like, rigid domains.
For decades, scientists have been trying to understand exactly how glass behaves, at the molecular level, as it approaches the transition temperature. Now a research group led by University of Michigan chemist Kevin J. Kubarych has applied ultrafast spectroscopy to observe the fastest molecular motions of a liquid hovering just above its glass transition temperature.
"Progress in demystifying the glass transition can have a wide impact in many other fields, including predicting optical and mechanical properties of polymers and understanding crowded cellular environments of living organisms," said Kubarych, an assistant professor of chemistry.
Working with U-M chemistry graduate students John King and Matthew Ross, Kubarych found that even on the time scale of picoseconds there are signatures of "dynamic arrest": The molecules become locked into their positions and long-range motion grinds to a near halt, though, structurally, the glass is indistinguishable from a liquid.
Typically, these effects are observed on much slower time scales of seconds, minutes, or even longer. A paper summarizing the research was published online April 9 in Physical Review Letters. King is the first author of the paper.
More information: Ultrafast α-Like Relaxation of a Fragile Glass-Forming Liquid Measured Using Two-Dimensional Infrared Spectroscopy
Sunday, April 01, 2012
New technique lights up the creation of holograms
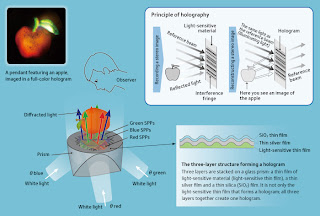
Subscribe to:
Posts (Atom)